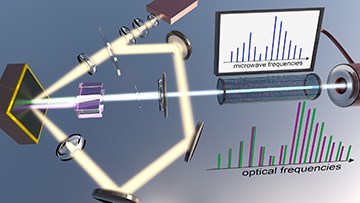
In the ETH Zurich team’s setup, the same laser pulse train, passed through a birefringent crystal, creates two mutually coherent, offset pulse trains, used to create a pair of optical frequency combs that can drive dual-comb spectroscopy of a gas sample. The dual-comb signal is picked up in the microwave domain using heterodyne detection. [Image: ETH Zurich/Sandro Link]
Scientists at ETH Zurich, Switzerland, have devised a way to generate a highly stable pair of offset frequency combs from a single, compact, free-running semiconductor disk laser. The team’s setup uses a birefringent crystal within the laser cavity to split the beam—with the frequency comb offset tunable simply by varying the crystal thickness. The approach, according to the team, “drastically reduces the complexity for dual-comb spectroscopy,” and could make that emerging technique practical for a wider range of industrial settings and applications.
Seeking stability
Frequency comb spectroscopy, which garnered the 2005 Nobel Prize in physics, uses laser-generated spectra containing hundreds of thousands or even millions of equally spaced, sharp lines as a ruler to precisely measure atomic and molecular species. In the dual-comb method, the addition of a second frequency comb to serve as a reference can dramatically boost the scan rate and spectral resolution of the method (see “Dual-comb spectroscopy,” OPN, January 2017). In both cases, the comb sources are generally femtosecond, mode-locked lasers, whose pulse trains in the time domain emerge as tightly spaced optical combs in the frequency domain.
A big challenge in bringing the dual-comb method into wide application, however, lies the demand for two mutually coherent, phase-stabilized pulse trains—achievable in the lab, but difficult using separate free-running lasers in industrial settings. Some dual-comb setups overcome that challenge using real-time, computational error correction to achieve the requisite stabilization between the two combs.
Another approach to creating two mutually coherent, stabilized frequency combs for dual-comb spectroscopy is to generate both from the same free-running laser. In such a setup, the two pulse trains that create the paired frequency combs share a single cavity, making the combs mutually coherent and stable without phase locking or post hoc error correction. Research teams have demonstrated single-laser, dual-comb setups with a number of lasers; one example, reported in 2016, used a ring cavity with counterpropagating laser modes, along with a nonlinear crystal, to create the offset pulse trains .
The MIXSEL way
The ETH Zurich team, led by OSA Fellow Ursula Keller, wanted to see if it could achieve a single-laser, dual-comb setup using an optically pumped semiconductor disk laser—a highly advantageous, compact platform based on wafer-scale technology, and thus a good potential candidate for mass production and wider application. In particular, the researchers zeroed in on a mode-locked, integrated external-cavity surface-emitting laser (MIXSEL) for their design. In a MIXSEL, the saturable absorber (for mode locking) and the gain medium are integrated into the same semiconductor chip; that chip forms the mirror at one end of a laser cavity, with an output coupler at the other end. An external diode laser pumps the MIXSEL cavity.
Within the MIXSEL cavity, the ETH Zurich team placed another element: a birefringent crystal. The crystal splits the MIXSEL source into two collinear pulse trains according to polarization; further, because of the optical path length difference of the two trains through it, the crystal also arranges for the required difference in pulse repetition frequency between the two pulse trains.
The result is two collinear optical frequency combs that can be interfered at a photodetector for dual-comb spectroscopy. As with other dual-comb setups, the actual measurement is taken using heterodyne detection, with the two THz-frequency combs mixed to create a single microwave-frequency comb; the mixed signal encodes the broadband, high-resolution spectroscopic signal of the two optical frequency combs into a beat frequency that’s readable using rapid, well-established RF digital signal processing.
A water test
The team tested the setup in a dual-comb spectroscopy experiment on water vapor, achieving rapid, good spectrographic results using a free-running laser with no active stabilization. Adding simple error-correction/stabilization of the mixed microwave comb improved the signal-to-noise characteristics and stability still further, according to the study. And the frequency difference between the two combs can be tuned by adjusting the thickness of (and, thus, the optical path length difference imposed by) the birefringent crystal.
One drawback of the system, according to the team, is that the MIXSEL-generated frequency combs have relatively restricted bandwidth. That means that analyzing molecular species with drastically different resonance frequencies (for example) may require different MIXSEL lasers.
Still, the researchers point out, because of the technology used, bandgap-engineering techniques that are well-established in the semiconductor industry could allow the center wavelength of these MIXSEL-based systems to be tailored relatively easily and cheaply. Thus an array of semiconductor disk lasers with specific operating wavelengths, the team suggests, could still prove more compact and cost-effective than a single expensive, broadly tunable Ti:sapphire laser. “We believe,” the study concludes, that “the dual-comb MIXSEL approach has the potential to bring dual-comb spectroscopy from a laboratory environment to the field for a wide range of industrial applications.”